ATPL Topic. Dive into Principles of Flight
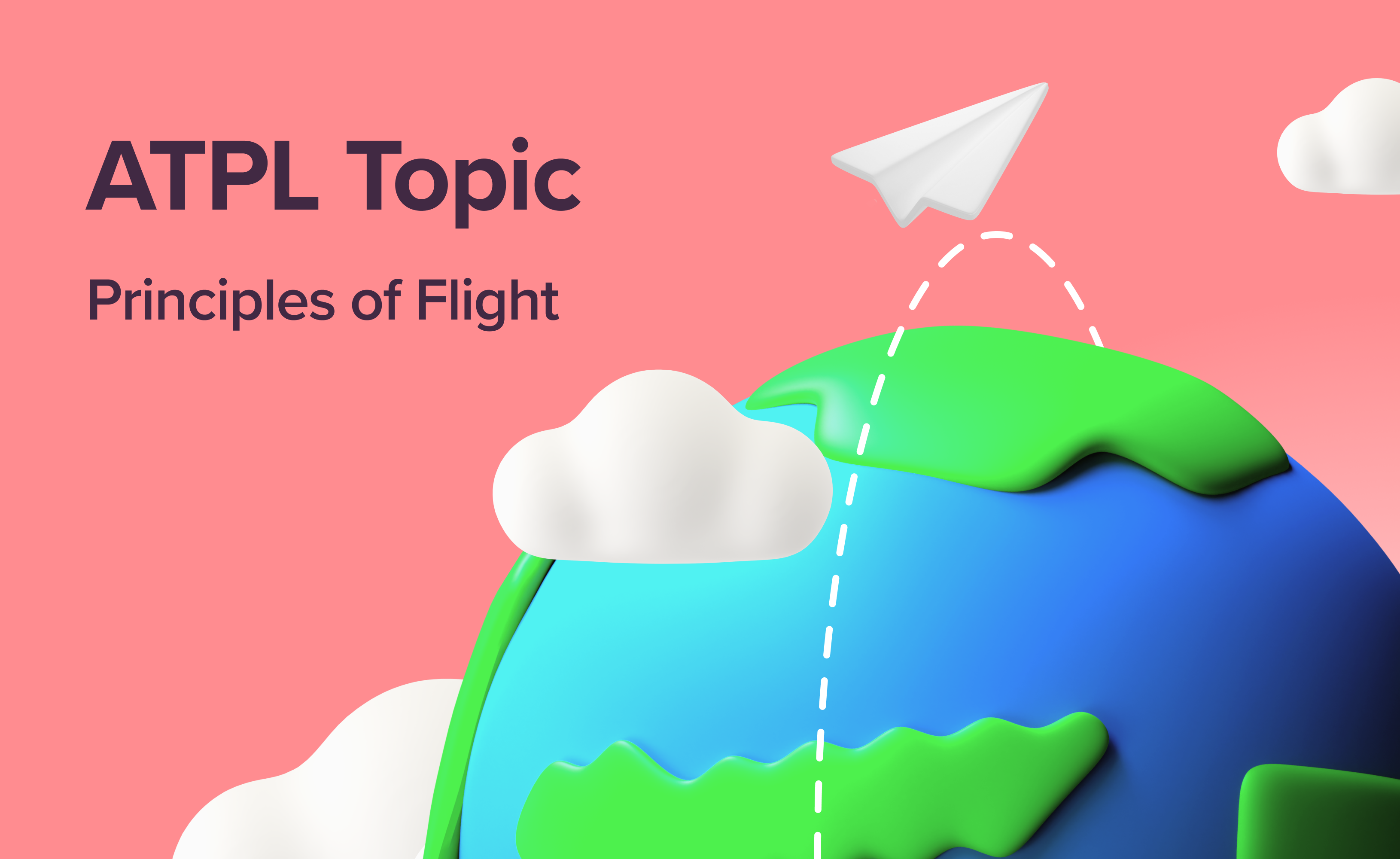
This ATPL subject earns a reputation among pilot students for its complexity, demanding maths skills and a deep comprehension of aerodynamic principles. Often deemed challenging, mastering this subject unlocks the key to comprehending aircraft performance, stability, and control — an essential skill set for any aspiring pilot.
This module lays the foundation for understanding how aircraft stay airborne and manoeuvre safely. Throughout this course of study, you delve into the key concepts that govern flight, including the four forces acting on an aircraft: lift, mass, thrust, and drag. By understanding these principles and mastering the calculations involved, you'll be well equipped to tackle more advanced topics later in your ATPL training.
Remember, a solid grasp of the fundamentals is vital for becoming a confident and competent pilot.
Introduction to the Principles of Flight
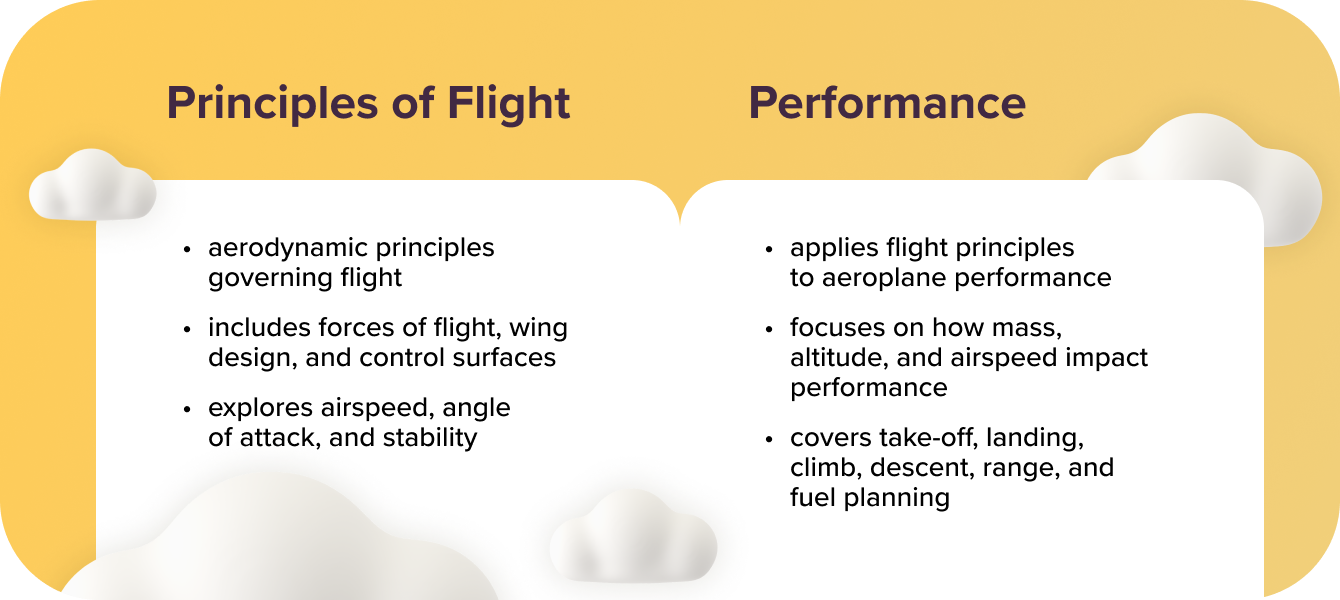
The Airline Transport Pilot Licence (ATPL) theoretical knowledge exam covers a wide range of subjects, including two critical areas: Principles of Flight (POF) and Performance (Aeroplane). While both subjects deal with how aeroplanes fly, they approach it from different angles. Let's delve into the key distinctions to solidify your understanding:
Principles of Flight (POF):
Focuses on the fundamental aerodynamic principles that govern how aircraft fly.
Covers concepts like the four forces of flight (lift, mass, thrust, drag), wing design, control surfaces, and how these elements work together to achieve flight.
Explores how factors like airspeed, angle of attack, and stability influence aircraft behaviour.
Lays the groundwork for understanding more advanced flight mechanics.
Performance (Aeroplane):
Builds upon the principles of flight by applying them to aircraft performance calculations.
Focuses on how different factors like mass, altitude, airspeed, and engine power impact an aircraft's performance.
Covers key concepts like take-off and landing performance, climb and descent rates, range and endurance, and fuel planning.
Equips pilots with the knowledge to calculate aircraft performance for various flight scenarios, ensuring safe and efficient operations.
Think of it like this. POF is the “why” behind flight — understanding the underlying scientific principles. Aeroplane Performance is the “how” of flight — applying those principles to calculate specific flight characteristics.
Both subjects are crucial for ATPL theoretical knowledge, as they provide a comprehensive understanding of how aircraft fly and perform under different conditions.
Exam Overview
Number of Questions: 46
Exam Duration: 1 hour 30 mins.
Difficulty: Hard.
76% of papers passed.
The Principles of Flight (POF) exam stands as a crucial milestone for those aiming to become Airline Transport Pilots (ATPL). Here's what you need to know.
The exam comprises 46 questions, demanding a strong grasp of key concepts. You'll have 1 hour and 30 minutes to complete the exam, so effective time management is crucial. The POF exam is considered challenging, requiring you to apply your knowledge to solve complex problems. Past results indicate a difficulty level rated as hard, with 76% of papers passed.
Please note that the minimum passing score for each ATPL exam is 75%; any score below this threshold, including 74.9%, results in a 'Fail'.
While the difficulty level might seem intimidating, the high pass rate reflects the value of dedicated study. Don't be discouraged! By focusing on understanding the core principles, practising calculations, and utilising effective study resources, you can confidently conquer the POF exam and take a significant step towards becoming a skilled pilot.
Subject Syllabus
This crucial exam delves into eight key sub-areas, each one a building block towards understanding the scientific principles that govern how aircraft fly. Let's break down each subtopic on the syllabus to get a clear picture of what you'll be learning:
081 01 Subsonic Aerodynamics
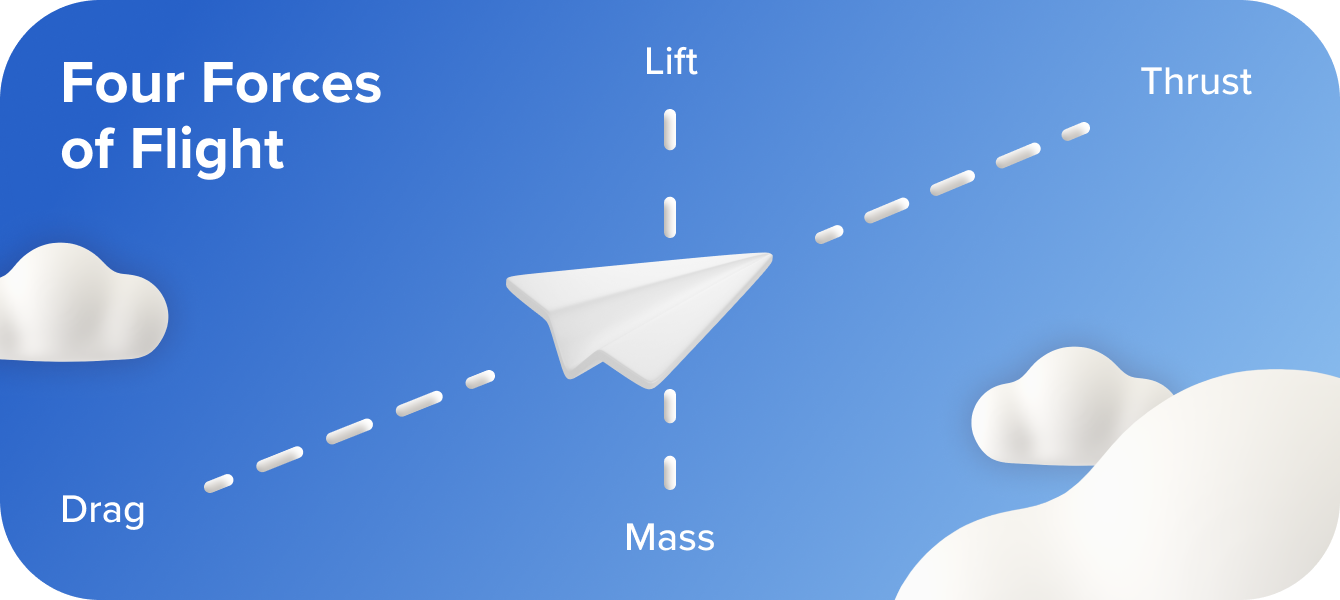
This is the foundation of understanding how aircraft fly at speeds below the speed of sound. You'll delve into the four forces of flight (lift, mass, thrust, drag) and how they interact with the aircraft's shape and movement. Mastering these principles will lay the groundwork for understanding more advanced concepts.
Four Forces of Flight in Action:
Lift
This is the opposing force to gravity, the one that keeps you airborne. The wing's shape plays a crucial role. As air flows over the curved upper surface of the wing, it creates lower pressure above the wing compared to the pressure below. This pressure differential generates lift, allowing the aircraft to counteract its mass and achieve flight.
Example: Ever notice how birds tilt their wings when turning? They're manipulating the angle of attack, which is the angle between the wing and the oncoming airflow. By increasing the angle of attack, they generate more lift for tighter turns.
Mass
This is the force that pulls the aircraft downwards due to gravity. It's influenced by the aircraft's mass (the amount of matter it contains) and the acceleration due to gravity.
Example: Aeroplanes have a maximum take-off weight (MTOW) limit. This considers the mass of the aircraft itself, fuel, passengers, and cargo. Exceeding this limit can compromise flight performance and safety.
Thrust
This is the propelling force that pushes the aircraft forward, generated by the engine(s). In simpler terms, it's what gets you moving! Thrust needs to be greater than drag for the aircraft to accelerate and maintain flight.
Example: During take-off, pilots adjust the engine power to generate sufficient thrust to overcome drag and propel the aircraft down the runway into flight.
Drag
This force acts in opposition to the direction of movement, essentially like air resistance. It's created by friction between the aircraft and the air, as well as the form drag caused by the aircraft's shape. It is important to reduce drag for efficient flight, even though it is inevitable.
Example: Have you seen aeroplanes retract their landing gear after take-off? This reduces drag significantly, allowing the aircraft to reach cruising altitude and fly more efficiently.
By understanding how these four forces interact with the aircraft's shape and movement in subsonic flight, you'll gain the foundation for mastering more advanced aerodynamic concepts.
081 02 High Speed Aerodynamics
Now, let's explore what happens when we push the envelope and fly at transonic and supersonic speeds. This section tackles the challenges encountered at high Mach numbers, including shockwaves, wave drag, and how aircraft design needs to adapt to maintain safe and efficient flight.
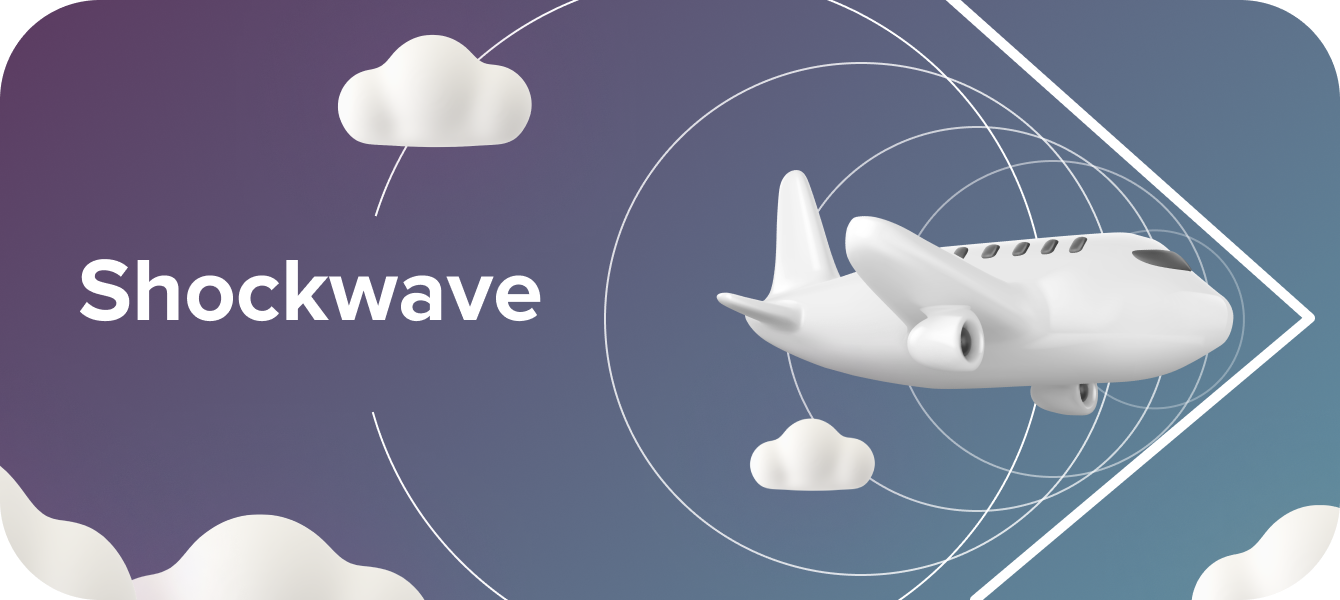
A New Set of Rules for Supersonic Speeds
At these higher Mach numbers, the familiar principles of subsonic aerodynamics encounter some fascinating disruptions.
Shockwaves
Imagine a sonic boom – that thunderous crack you hear when an aircraft breaks the sound barrier – shockwaves cause it. As the aircraft approaches the speed of sound, the air molecules ahead can't get out of the way fast enough, creating a pressure wave that propagates outward like a ripple in a pond. These shockwaves can cause significant drag and buffet the aircraft, making control more challenging.
Wave Drag While drag exists at all speeds, it increases dramatically at transonic and supersonic speeds due to the formation of shockwaves. This “wave drag” can significantly reduce an aircraft's efficiency and limit its range.
Adapting for Supersonic Flight:
Aircraft designers have developed ingenious solutions to overcome these challenges and enable safe, efficient supersonic flight. Here are some key adaptations:
Swept Wings
Unlike the straight wings of subsonic aircraft, supersonic aeroplanes have swept wings. This design angles the leading edge of the wing backwards, delaying the formation of shockwaves and reducing wave drag at higher speeds.
Slender Fuselage
Imagine a dart cutting through the air. Supersonic aircraft often have slender fuselages to minimise drag by reducing the cross-sectional area exposed to the oncoming airflow.
Variable Geometry Wings
Some advanced aircraft utilise variable geometry wings, where the sweep angle of the wings can be adjusted during flight. This allows for optimal performance at both subsonic and supersonic speeds.
As you progress through your ATPL training, this knowledge will be crucial for understanding the capabilities and limitations of different aircraft types and making informed decisions in various flight scenarios.
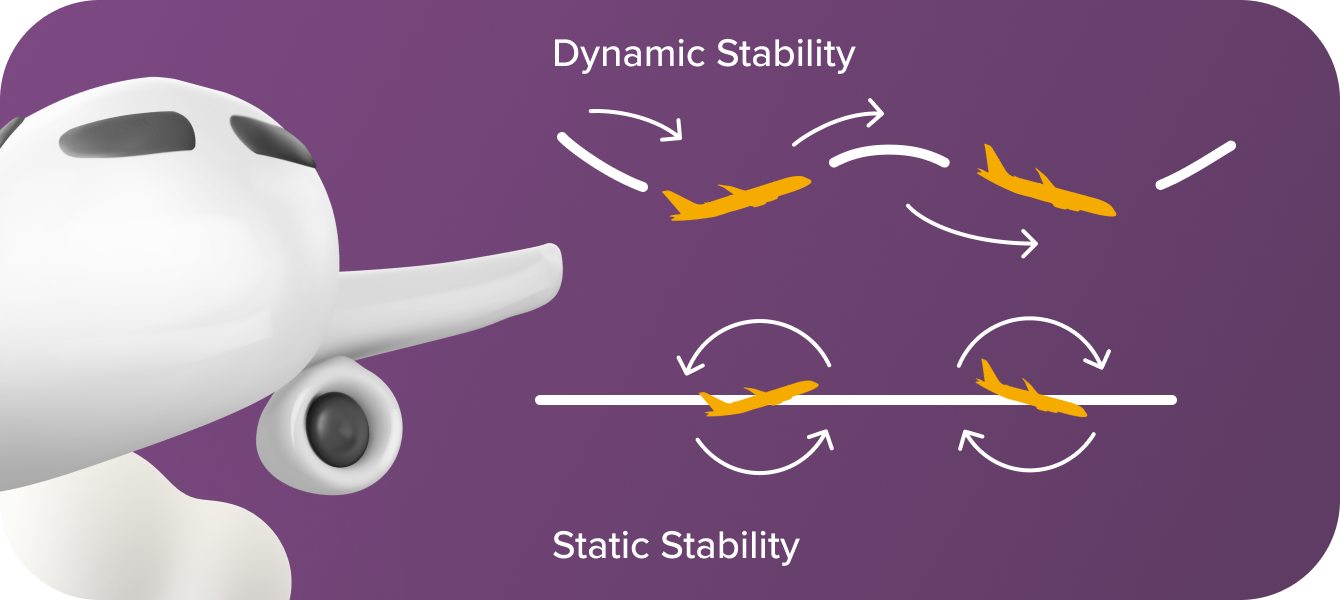
081 03 Stall, Mach Tuck and Upset Prevention and Recovery
This critical topic focuses on recognising and recovering from situations where an aircraft loses lift (stall) or experiences Mach tuck (sudden loss of control at high speeds). You learn how to identify the signs, understand the causes, and most importantly, master the techniques to regain control and ensure flight safety.
The Dreaded Stall
A stall occurs when an aircraft loses sufficient lift to maintain level flight. This can happen at any airspeed if the angle of attack (the angle between the wing and the oncoming airflow) becomes too high. The wing can no longer generate enough lift to overcome the aircraft's body mass, leading to a descent.
Identifying a Stall. The aircraft may experience a buffeting sensation, a shuddering caused by airflow separation over the wing. The aircraft will start to descend as it loses lift. While airspeed may seem high, the indicated airspeed (IAS) may not be reflecting the true airspeed (TAS) due to the stall. Modern aircraft have stall warning systems that activate with audible or visual cues to alert the pilot.
Recovery from a Stall. The key to stall recovery is reducing the angle of attack and regaining lift. This is achieved by lowering the nose of the aircraft to increase airspeed over the wings. Once sufficient airspeed is regained, the aircraft will recover from the stall and level flight can be re-established.
Mach Tuck
Mach tuck is a phenomenon encountered at high speeds (usually transonic or supersonic) when shockwaves form around the wings and disrupt airflow. This can lead to a sudden loss of control, particularly a pitch-down moment, causing the nose of the aircraft to drop abruptly.
Identifying Mach Tuck. The aircraft may experience a sudden, unexpected nose-down pitch. The pilot may experience a temporary loss of elevator control due to the disrupted airflow.
Recovery from Mach Tuck. Recovery from Mach tuck involves reducing the airspeed by easing off engine power and allowing the aircraft to accelerate below the critical Mach number where the shockwaves start to form. Once the shockwaves dissipate, the pilot will regain control.
Upset Prevention and Recovery (UPRT)
Aircraft upsets can occur for various reasons, including turbulence, wind shear, or pilot error. These upsets can cause the aircraft to deviate from its intended flight path and attitude.
Upset Prevention & Recovery techniques focus on developing situational awareness, recognising the signs of an upset, and applying appropriate control inputs to regain control of the aircraft and maintain flight safety. This link has related information about the UPRT.
By mastering these critical skills and understanding the underlying principles of stall, Mach tuck, and upset prevention & recovery, you'll be well-equipped to handle unexpected situations and ensure your safety and the safety of your passengers. Remember, an ounce of prevention is worth a pound of cure; practising these techniques in a simulator environment can make a world of difference in real-world situations.
081 04 Stability
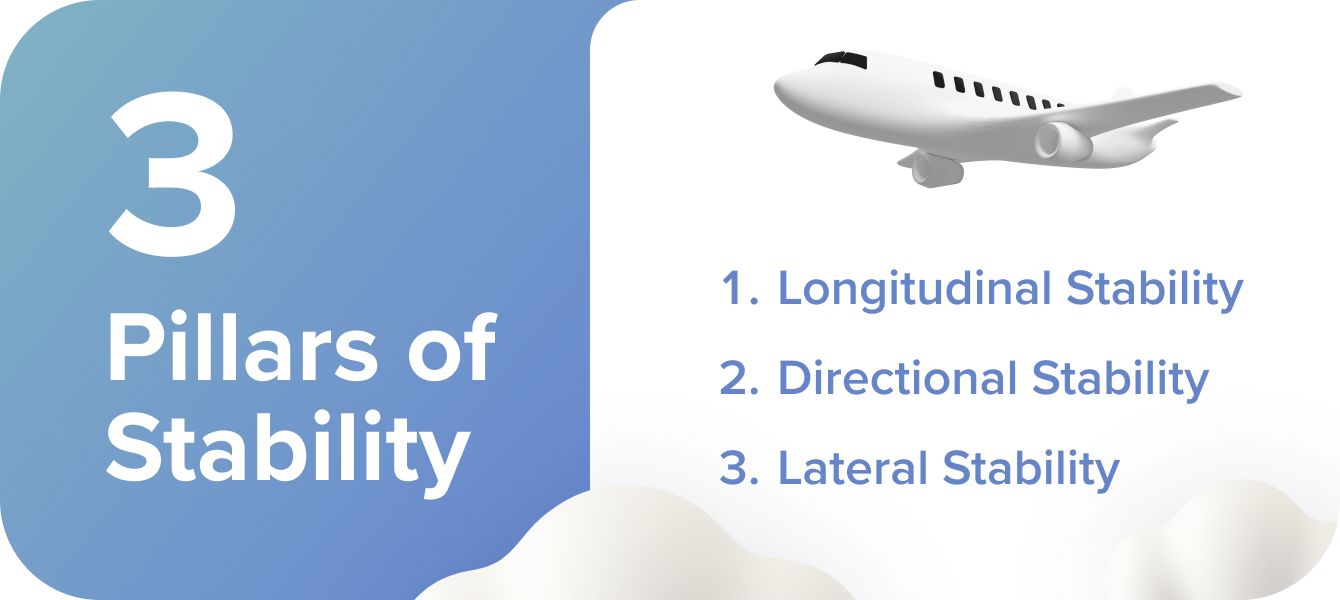
Imagine an aircraft gliding smoothly through the air. This is all about stability! This section explores how various design elements and pilot inputs contribute to an aircraft's ability to maintain its desired attitude and heading. You learn about different types of stability (longitudinal, directional, etc.) and how they impact flight control.
3 Pillars of Stability
An aircraft's stability can be likened to a three-legged stool. Each leg represents a different type of stability, and all three need to function together for safe and efficient flight:
1. Longitudinal Stability
This refers to the aircraft's ability to maintain its pitch attitude (nose up/down) without constant pilot input. Imagine a seesaw – a longitudinally stable aircraft will naturally return to level flight after a slight pitch disturbance, just like a balanced seesaw return to the centre position. Factors influencing longitudinal stability include wing design, centre of gravity (CG) location, and the lift control surface.
Example: If an aeroplane encounters a sudden gust of wind that pitches the nose up, a longitudinally stable aircraft will automatically generate aerodynamic forces that pitch the nose back down, returning the aircraft to its original pitch attitude.
2. Directional Stability
This focuses on the aircraft's ability to maintain its heading (yaw left/right) without constant pilot input. Think of a weather vane – a directionally stable aircraft will naturally return to its original heading after a slight yaw disturbance, just like a weather vane turns back to face the wind. Factors influencing directional stability include the vertical stabiliser (fin) and rudder control surface.
Example: If an aeroplane encounters a crosswind that yaws it to the right, a directionally stable aircraft will automatically generate aerodynamic forces that counter the yaw and nudge the nose back towards the original heading.
3. Lateral Stability
This concerns the aircraft's ability to maintain its roll angle (wing level) without constant pilot input. Imagine a bicycle – a laterally stable aircraft will naturally return to wings level after a slight roll disturbance, just like a bicycle tends to stay upright when moving forward. Factors influencing lateral stability include dihedral angle (upward angle of wingtips) and ailerons (control surfaces for roll).
Example: If an aeroplane encounters turbulence that causes a wing to dip (roll), a laterally stable aircraft will automatically generate aerodynamic forces that lift the lowered wing and restore level flight.
This knowledge of aircraft stability forms the foundation for understanding more advanced flight control techniques and manoeuvres. As you progress through your ATPL training, you'll discover how pilots rely on various control inputs and automation systems to maintain stability in challenging conditions, ensuring a safe and enjoyable flying experience.
081 05 Control

Imagine yourself piloting a majestic aeroplane, gracefully manoeuvring it through the skies. This feat is made possible by a remarkable system of flight controls, the very tools that translate pilot inputs into precise aircraft movements.
An aeroplane relies on a sophisticated interplay of moveable surfaces on its wings and tail to achieve controlled flight. Let's meet the key players in this area:
Ailerons: Hinged control surfaces located on the trailing edges of the wings. By deflecting the ailerons in opposite directions (one aileron up, the other down), pilots control the aircraft's roll (banking). Imagine raising the right aileron – this increases drag on the right wing and increases lift on the left wing, causing the aircraft to roll to the right and initiate a turn.
Elevators: Hinged on the horizontal stabiliser (tailplane), elevators are responsible for controlling the aircraft's pitch (nose up/down). Raising the elevators deflects them upwards, pushing the tail down and causing the nose to pitch up for climbing. Conversely, lowering the elevators deflects them downwards, pushing the tail up and causing the nose to pitch down for descent.
Rudder: This hinged surface located on the vertical stabiliser (fin) is used to control the aircraft's yaw (nose left/right). Deflecting the rudder to the right pushes the tail right, causing the nose to yaw right and initiating a coordinated turn. The rudder works in conjunction with the ailerons for smooth and efficient turning manoeuvres.
081 06 Limitation
Unlike a car that can theoretically keep driving as long as there's fuel in the tank, every aircraft operates within a specific set of limitations. These limitations, established through rigorous testing and certification, ensure safe operation and prevent exceeding the aircraft's structural capabilities.
Here are some key limitations and how they impact flight planning and decision-making:
Maximum Take-Off Weight (MTOM and/or MTOW.): Exceeding MTOW can compromise performance, increase take-off distance, and place undue stress on the aircraft's structure. Pilots meticulously calculate weight and balance figures during flight planning to ensure they stay within the MTOW limit for a safe take-off. We all say mass when we mean weight, and vice-versa. So expect to see MTOM and/or MTOW.
Maximum Operating Speed (VMO): VMO considers factors like engine limitations, controllability, and airframe stress. Flying above VMO can lead to structural damage or control issues. Pilots factor VMO into their flight plans to ensure they remain within safe speed limits throughout the flight.
G-Force Limitations: When an aircraft manoeuvres, it experiences g-forces, which are multiples of the force of gravity acting on the aircraft and its occupants. Aircraft have positive and negative g-force limitations.
Other Limitations: There are additional limitations to consider, such as maximum landing weight, minimum control speeds, and engine limitations. Pilots take into account all these limitations in their flight planning and decision-making.
081 07 Propellers
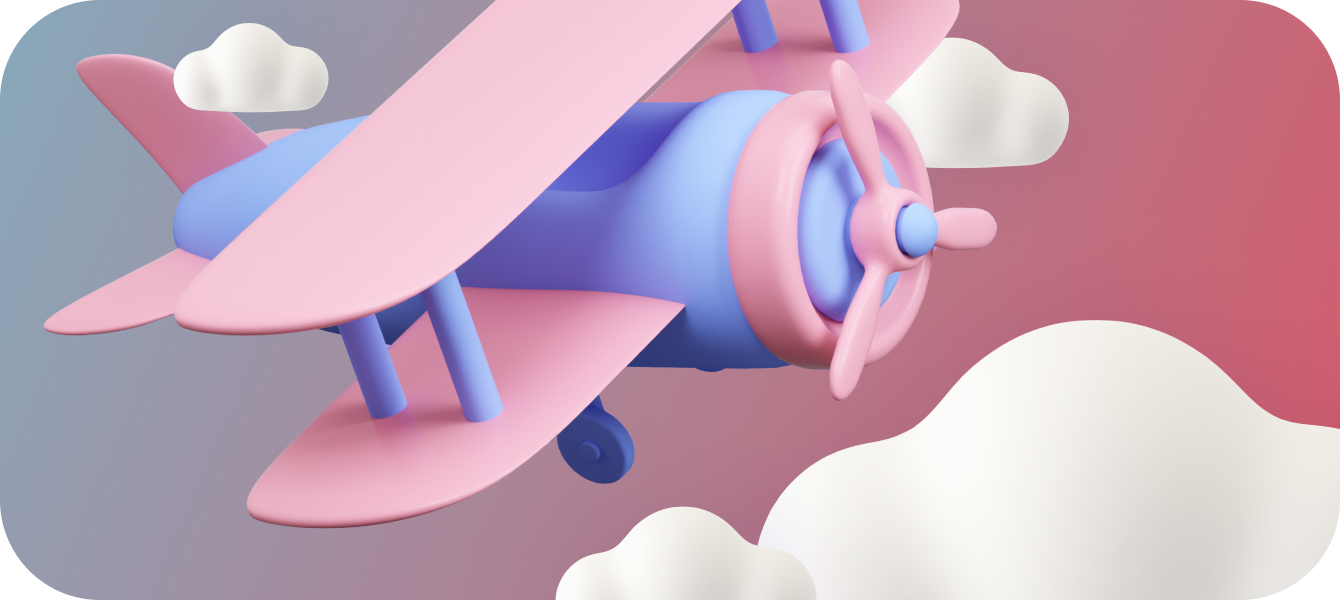
For those of you aspiring to master the world of propeller-driven aircraft, this section delves into the fascinating world of propellers – the workhorses that convert engine power into thrust, propelling the aircraft forward.
Imagine a propeller as a spinning wing. As the blades rotate, they create lift, but unlike a wing that generates lift perpendicular to its motion, a propeller's blades are angled to “bite” into the air, pushing it backwards. This backward airflow creates a reaction force, propelling the aircraft forward according to Newton's Third Law of Motion (for every action, there is an equal and opposite reaction).
Propeller design plays a crucial role in performance. The number and shape of the blades can influence factors like thrust, efficiency, and noise. Two-blade propellers offer simplicity but may be less efficient than three or four-blade designs. Blade shape also plays a role, with wider blades generating more thrust but potentially creating more drag at higher speeds.
081 08 Flight Mechanics
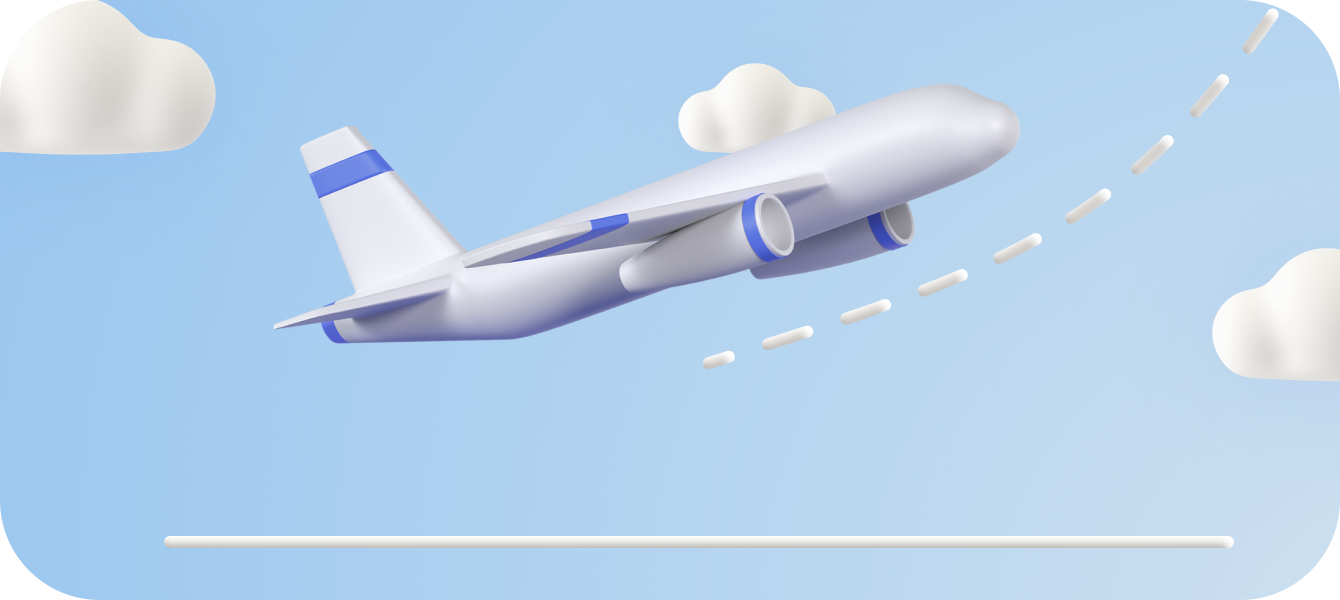
This section ties everything together! Here, you take all the knowledge you've accumulated – the forces of flight, control surfaces, aircraft limitations – and apply them to understand how an aircraft performs under different conditions. You'll learn how factors like mass, altitude, engine power, and control inputs impact flight characteristics like take-off, climb, descent, and cruise performance.
Mass. A heavier aircraft requires more thrust to overcome its inertia and achieve the desired performance during take-off, climb, and manoeuvrability.
Altitude. As you climb, air density decreases, reducing the efficiency of both the propeller and the wings. This means you'll need to adjust engine power or airspeed to maintain performance at higher altitudes.
Engine Power. Managing engine power is crucial for optimal performance throughout the flight. Pilots learn to adjust power settings for take-off, climb, cruise, descent, and landing.
Control Inputs. The pilot's use of control surfaces (ailerons, elevators, rudder) directly affects flight characteristics. Understanding how these inputs influence aspects like roll, pitch, and yaw allows for precise manoeuvring during various flight phases.
You'll gain a profound understanding of how an aircraft flies by mastering these interrelationships between aircraft design, environmental factors, engine power, and control inputs. This knowledge will be instrumental in making informed decisions, operating the aircraft safely, and ultimately, achieving your dream of soaring through the skies!