It's All Connected: TWR, Drag, and the Climb Rates
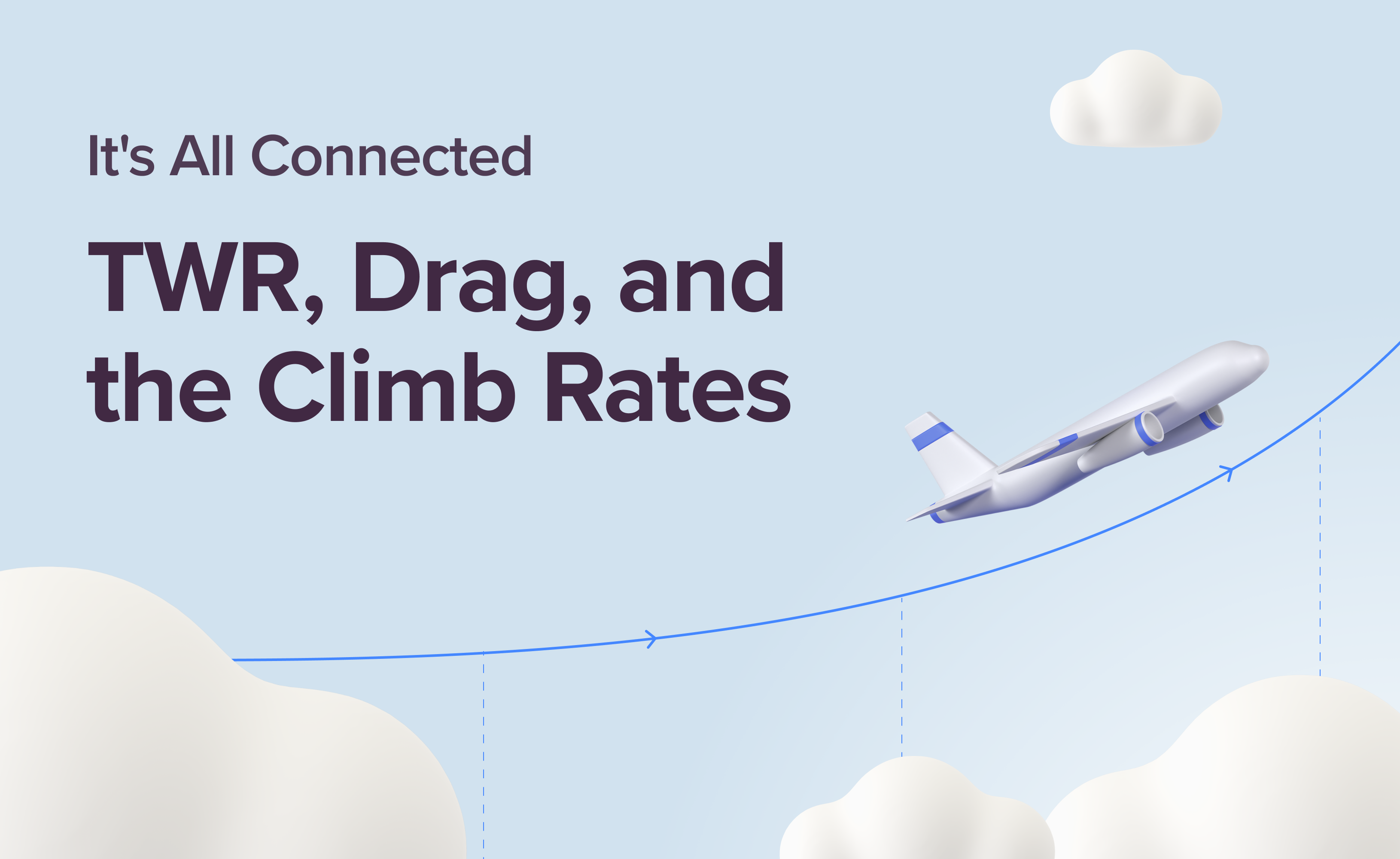
Why do some aircraft leap into the sky like rockets while others take their time to climb? Or why does one aircraft need a longer runway, while another lifts off effortlessly in half the distance?
The answer lies not in just one factor but in a web of interconnected forces that determine how an aircraft performs in flight — especially during climb and descent. At the heart of this web are three major players: Thrust-to-Weight Ratio (TWR), Drag, and the aircraft’s Rate of Climb (RoC) or Descent (RoD).
Understanding how these forces work together can transform the way you think about aircraft performance. Whether you're just beginning your flight training or brushing up on theory, this blog breaks down the complex relationships between TWR, drag, size and how they all come together to influence performance speeds like Vx and Vy.
We'll connect the dots between power, force, aircraft size, and aerodynamics — making what might seem like a dry technical topic not only clearer but intuitive.
Let’s dive in and uncover how it’s all connected in the world of flight.
What Is Aircraft Performance?
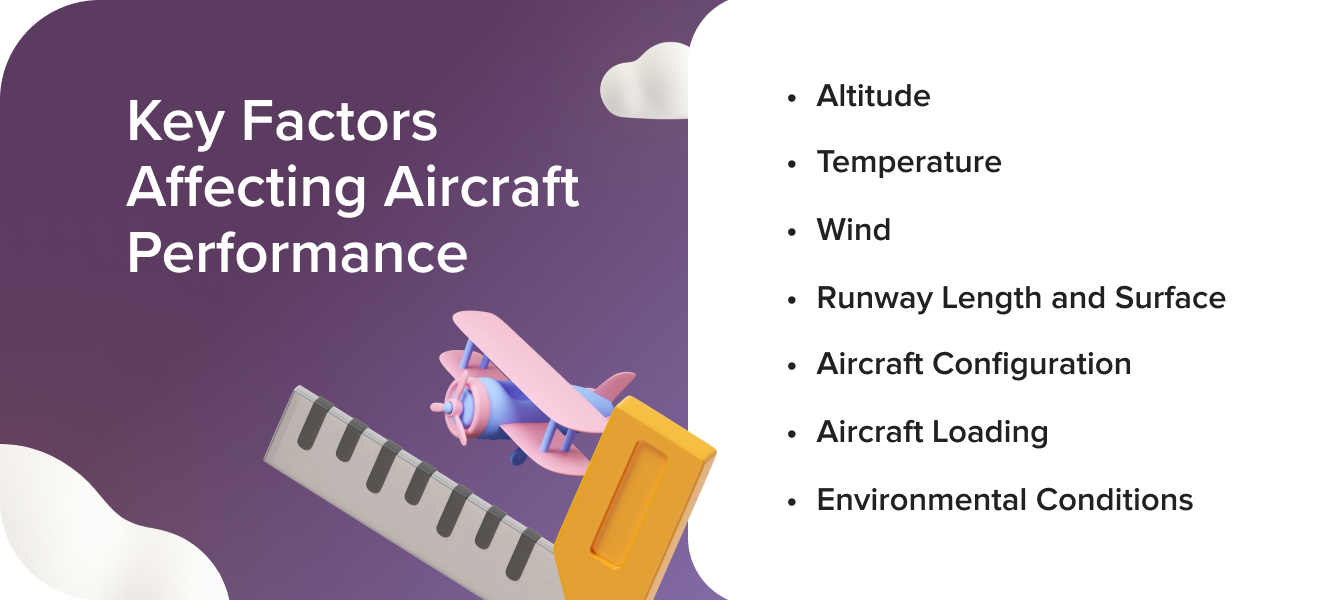
Aircraft performance refers to how well an aircraft can operate under various conditions — from take-off and climb to cruise and landing. It’s one of the 13 ATPL (Airline Transport Pilot Licence) exam subjects and is essential knowledge for any pilot aiming to master how environment, loading, and aircraft setup impact flight. Key factors influencing performance include altitude, temperature, wind, runway conditions, aircraft configuration, loading, and broader environmental conditions.
Explore key subtopics & core concepts of the Performance Syllabus.
We’ll focus especially on how aircraft configuration affects performance — diving into how changes in drag, thrust, weight, and setup influence critical speeds like Vx, Vy, and climb/descent rates.
Thrust-to-Weight Ratio (TWR)
The Foundation of Performance
Thrust-to-Weight Ratio (TWR) is the first step in understanding aircraft performance. This simple yet powerful metric compares the thrust produced by the engines to the total weight of the aircraft — and it tells us a lot about what the aircraft is capable of in the air.
TWR = Thrust / Weight
Think of it as a performance pulse:
If TWR > 1, the aircraft can generate more thrust than it weighs. That means it can accelerate vertically, climb faster, and respond more energetically to control inputs — an ideal scenario for fighter jets and high-performance aircraft.
If TWR < 1, the aircraft won’t be able to overcome its weight using thrust alone. It can still climb, but it relies on forward motion and lift — typical of commercial jets and general aviation aircraft.
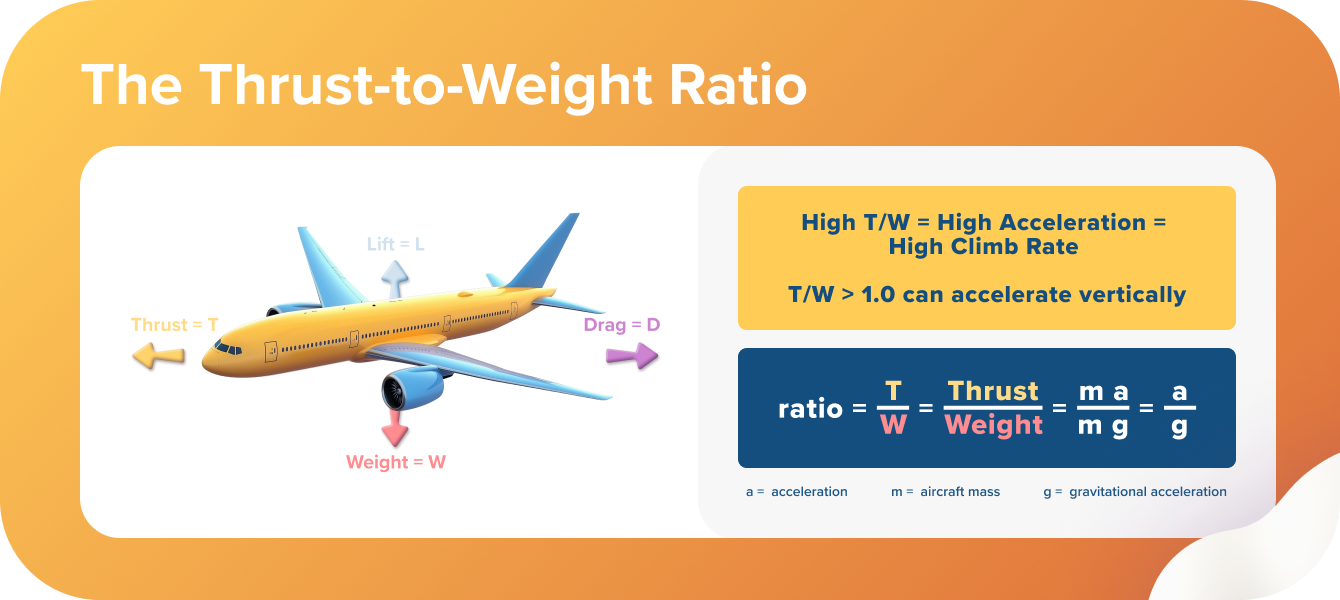
Some modern military jets like the F-22 Raptor have a TWR well above 1.0, allowing them to perform vertical climbs and high-speed manoeuvres that most aircraft could only dream of.
As shown in the visualisation, TWR links directly to how quickly an aircraft can accelerate and climb. The higher the TWR, the greater the potential Rate of Climb (RoC) — making it a crucial factor in flight dynamics and mission planning.
Understand the fundamental forces of lift, drag, thrust, and gravity that govern the magic of flight in our blog Mastering Flight: The 4 Forces Explained.
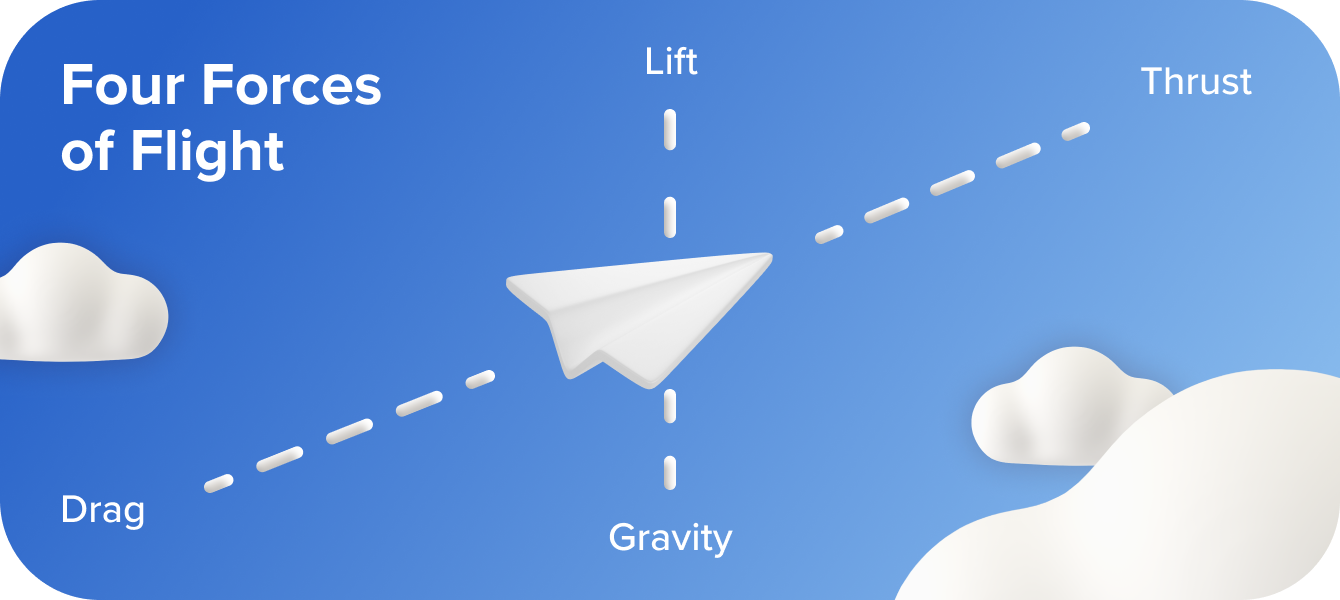
Rate of Climb & Rate of Descent
The Vertical Game
Now, let's shed light on what decides how quickly an aircraft can soar skyward — or descend smoothly back to earth. It’s not just about pulling the yoke or easing off the throttle. The answer lies in the dynamic relationship between Thrust-to-Weight Ratio (TWR), Drag, and airmanship.
At its core, the Rate of Climb (RoC) or Rate of Descent (RoD) reflects an aircraft’s vertical speed — how fast it gains or loses altitude over time.
Let’s break it down.
Climbing Higher: A higher TWR means the engines are packing more punch relative to the aircraft’s weight. That leads to more excess thrust (thrust minus drag) — and that excess is what gives the aircraft the muscle to accelerate upward. The more extra thrust you’ve got, the faster you can climb.
Levelling Out: When thrust only equals drag, the aircraft maintains level flight. There’s no upward force left to fuel a climb.
Coming Down: While TWR is mostly about climbing, it also plays a subtle but important role in descent control. In powered descents, pilots can adjust throttle settings to tweak the thrust — higher thrust means a slower descent, and lower thrust means a steeper one. Aircraft with higher TWRs offer more flexibility in managing descent patterns.
In Glide (Unpowered Descent): If thrust is gone (say, engine failure), descent performance shifts focus to the lift-to-drag ratio (L/D). But even then, weight matters. Heavier aircraft (with the same L/D) descend faster, so TWR still echoes in the background, influencing the glide behaviour.
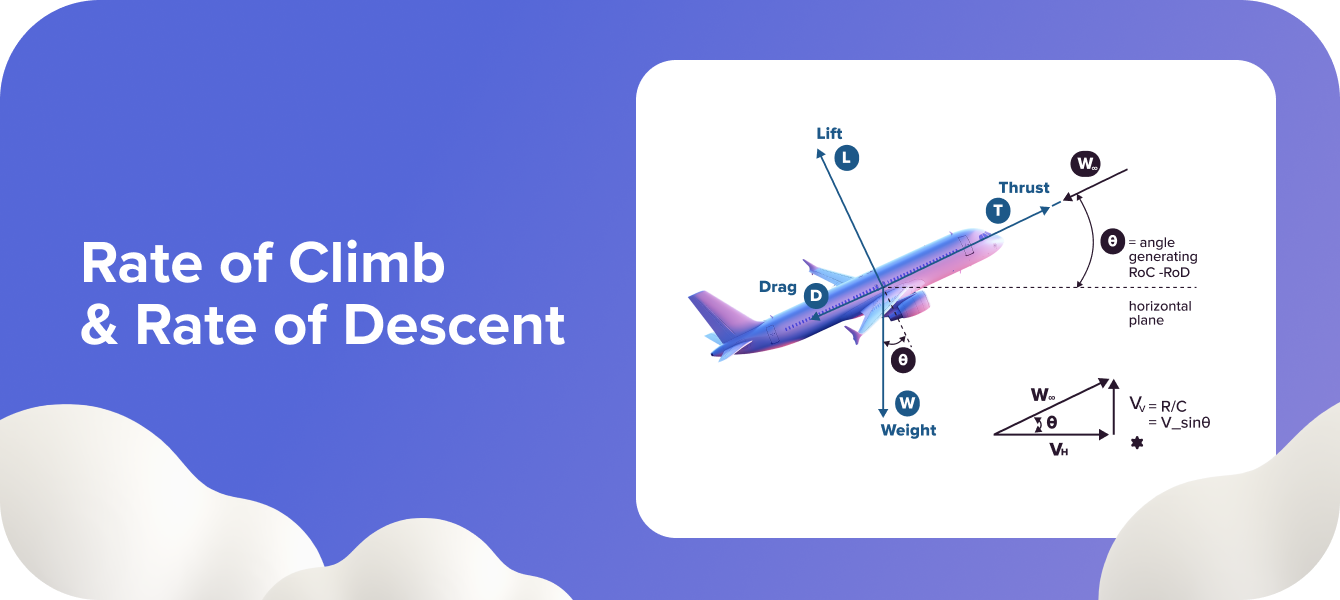
Key Equation in the Visual Vv = V⋅sin(θ) – where VvV is vertical speed and θ is the flight path angle relative to the horizon. Steeper angles = greater RoC or RoD.
Consistently achieve smooth landings and master flap adjustments with our in-depth guide: Flaps, Lift & Drag: The Secret to Smooth Landings.
Size Effects on Drag
When Bigger Isn’t Always Better
As aircraft grow in size, their aerodynamic profile doesn’t just scale — it transforms. And one of the biggest transformations? Drag.
Why Bigger Means More Drag: Larger aircraft have greater surface areas, which increases skin friction drag — the resistance created as air flows over the aircraft’s body. Their frontal area also expands, amplifying form drag — the air resistance from the shape pushing through the air. Together, these effects mean one thing: more total drag.
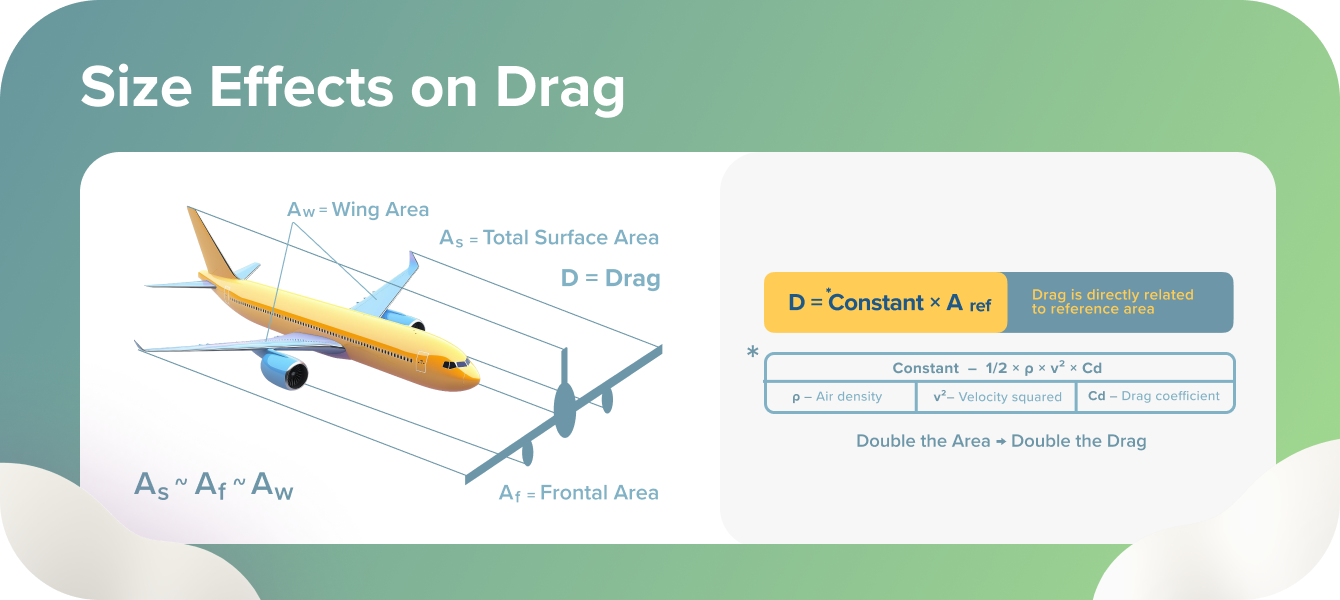
Key Equation in the Visual Drag increases proportionally with reference area:
D = Constant × Aref – where Сonstant – 1/2 × ρ × v² × Cd
So, double the area = double the drag, assuming all else stays the same.
Impact on Climb: To keep climbing, an aircraft needs excess thrust (thrust that goes beyond just cancelling out drag). But when drag increases due to size, more of the available thrust gets eaten up, leaving less for vertical acceleration. That means a lower Rate of Climb (RoC) — even if the aircraft’s Thrust-to-Weight Ratio (TWR) remains the same.
Impact on Glide: In an unpowered glide, high drag causes a steeper glide angle, reducing how far the aircraft can travel for each foot of altitude lost. The result? A higher Rate of Descent (RoD) — especially noticeable at the same airspeed compared to a sleeker, smaller aircraft.
Double the size, and you double the drag — but your engines don’t magically get twice as strong. More drag means less thrust left for climbing and a steeper descent if the power goes out.
Gain a deeper understanding of how aeroplanes turn, pitch, and roll. Learn about the 3 Axes of Rotation in our latest blog.
Vx and Vy
The Relationship Between Force and Power
Now that we've explored how TWR, drag, and aircraft size interact to shape climb performance, let’s bring it all together. These factors also play a crucial role in determining two of aviation's most important performance speeds: Vx and Vy.
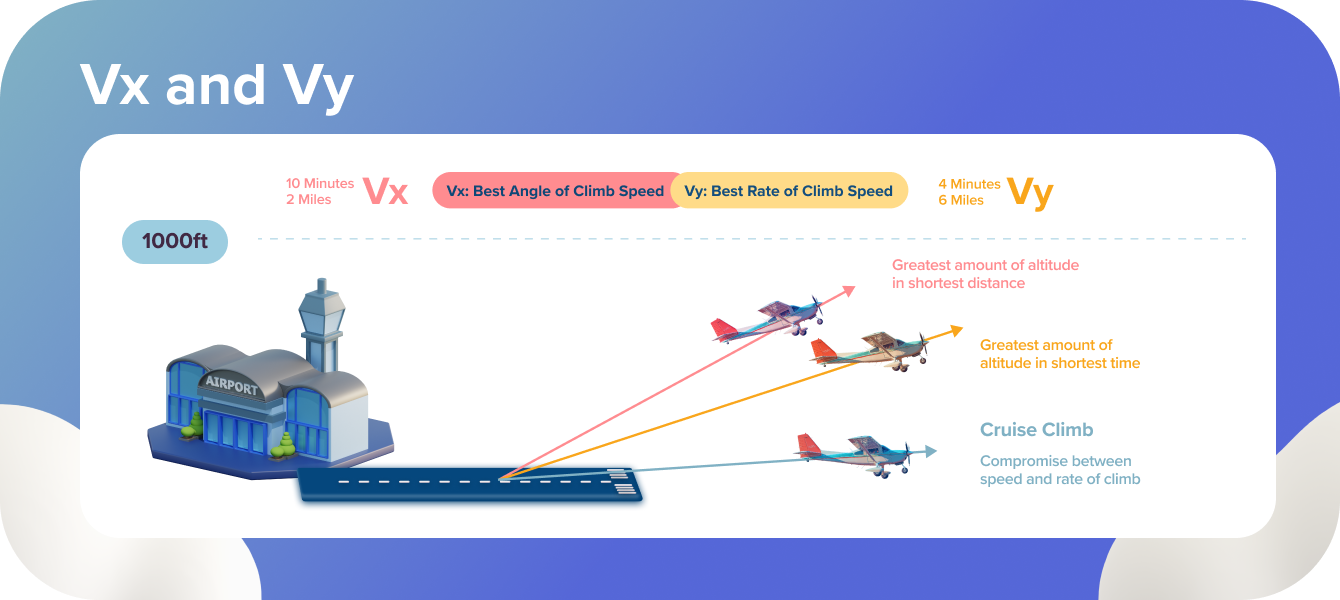
Vx – Best Angle of Climb Speed If your mission is to clear an obstacle with minimal horizontal distance — think mountain ridges or short runway departures — Vx is the speed to fly. It gives you the maximum altitude gain over the shortest horizontal distance. It happens at the speed where excess thrust (force) is greatest — i.e., the most push available after overcoming drag. Because thrust is a force, Vx is fundamentally about optimising the aircraft’s force balance.
In the visual: The steepest path (red) reaches 1000 ft in 2 miles and 10 minutes.
Vy – Best Rate of Climb Speed On the other hand, if your goal is to reach cruising altitude as quickly as possible, Vy is your best bet. It provides the greatest altitude gain over time and is reached where excess power (force × velocity) is maximised. Since power equals force multiplied by velocity, Vy reflects the point where your engine’s energy output most efficiently translates into climbing — or gaining potential energy fast. It’s all about how quickly the aircraft gains energy to climb — Vy occurs at a higher airspeed than Vx.
In the visual: The most time-efficient climb (yellow) reaches 1000 ft in 4 minutes over 6 miles.
Cruise Climb Speed
A compromise between Vx and Vy — balancing visibility, cooling, fuel efficiency, and passenger comfort.
Do you know the difference between true altitude and pressure altitude? Find out the answer and learn about other types of altitude in our blog: Altitude Basics: 5 Types Of Altitude Explained.
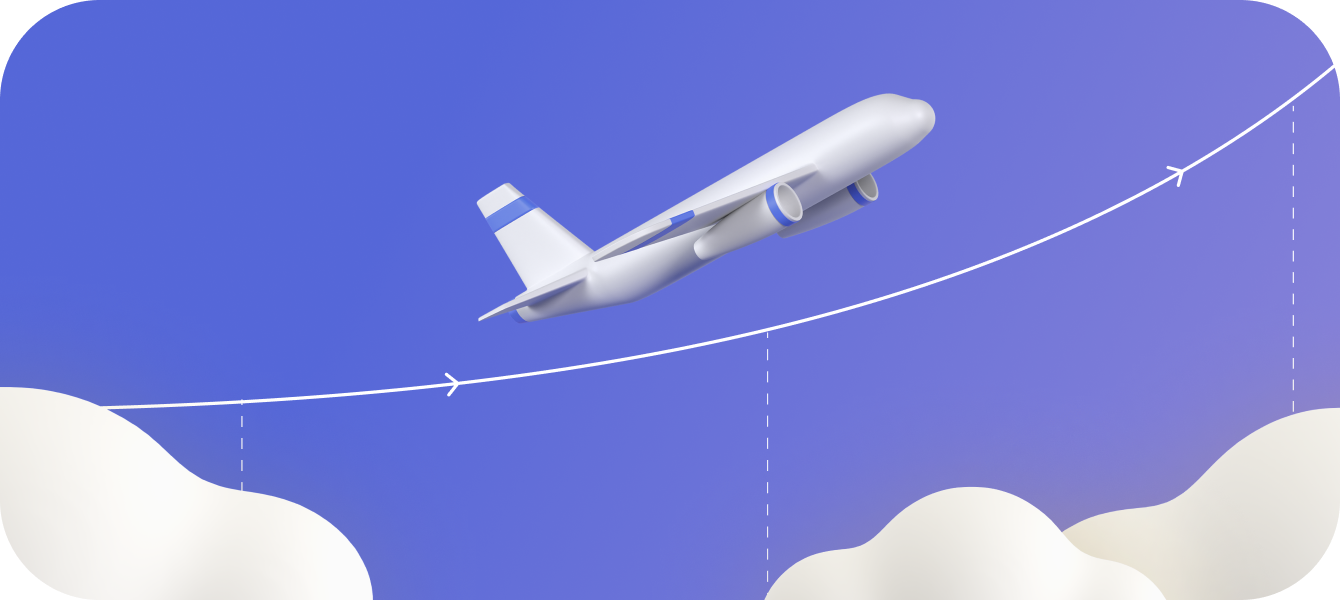
Airhead's Takeaway
The TWR sets the fundamental limit on how much excess thrust is available for climb.
Size effects on drag influence the amount of thrust required to overcome air resistance. Higher drag reduces the excess thrust available for climb, directly impacting the Rate of Climb.
Vx is the speed that leverages the excess thrust to achieve the steepest climb angle.
Vy is the speed that leverages the excess power to achieve the fastest rate of altitude gain.